Long-Chain Polyunsaturated Fatty Acids Supplementation and Respiratory Infections
Key insights
Long-chain polyunsaturated fatty acids (LCPUFAs) are essential for the maintenance and optimal functioning of
immune cells. The metabolites of both omega-3 and omega-6 fatty acids play an important role in the synthesis of immune mediators, such as prostaglandins, leukotrienes, thromboxanes, protectins, and resolvins. LCPUFAs are
synthesized by elongation and desaturation of the carbon chain from the essential PUFAs, such as linoleic acid (LA) and alpha linolenic acid (ALA). In the current pandemic, great attention has been paid to immune modulation, with the ultimate goal of infection prevention and treatment of COVID-19 patients.
Current knowledge
There are few clinical trials on LCPUFA supplementation for the prevention and management of respiratory tract infections (RTIs). This narrative review aimed to identify whether LCPUFA supplementation was effective at protecting against RTIs. Eight clinical studies were analyzed, 5 of which were conducted in children (aged 0–11 years) and 3 in adults (aged 18–45 years). A total of 4,107 subjects were included. A variety of LCPUFA types and dosages were used for the supplementations, ranging in duration between 4 and 9 months.
Practical implications
The available results point towards a potential beneficial role of LCPUFA supplementation in the prevention of RTIs. In children, the protective effects were reflected by a lower number of infectious episodes, reduced symptoms, and reduced school absences. Positive results were seen with supplemented formula and dietary supplements in the pediatric population. However, some studies have yielded inconclusive findings. The heterogeneity of study design and inclusion criteria are major limitations in our ability to translate these findings into concrete recommendations that benefit patients. Randomized controlled trials designed and powered to address questions on dose, duration,
and outcomes of LCPUFA on RTIs are still needed.
Recommended reading
Imhoff-Kunsch B, Stein AD, Martorell R, Parra-Cabrera S, Romieu I, Ramakrishnan U. Prenatal docosahexaenoic acid supplementation and infant morbidity: randomized controlled
trial. Pediatrics. 2011;128(3):e505–12.
Key Messages
• Long-chain polyunsaturated fatty acids (LCPUFAs) and their metabolites are involved in the control of chronic and acute inflammations.
• No data were available on the role of LCPUFAs in COVID-19 disease.
• More focused randomized controlled trials are necessary to evaluate the effect of LCPUFA supplementation.
Abstract
Background: Long-chain polyunsaturated fatty acids (LCPU- FAs) can actively affect the maintenance and optimal functioning of immune cells. The metabolites of both omega-3 and omega-6 play an important role in the synthesis of different mediators, such as prostaglandins, leukotrienes, thromboxanes, protectins, and resolvins, that can interfere with the virus and modulate inflammation.
Summary: In this narrative review, we aim to identify whether LCPUFA supplementation may be effective in protecting the population against respiratory tract infections. We included only randomized controlled trials performed in both pediatric and adult subjects. Eight papers were selected: five trials were conducted in a pediatric population and three in adults. Different concentrations of fatty acids supplementation were associated with a lower incidence of common respiratory symptoms, except for two studies that did not provide significant results. Most of the studies are of low quality, and respiratory infections were assessed as secondary or even safety outcomes.
Key Messages: No data were available on the role of LCPUFAs in coronavirus disease 2019 (COVID-19). Although these data showed that LCPUFAs may be effective in preventing respiratory tract infections, future studies are still needed to clarify their possible co-adjuvant role in the prevention and treatment of respiratory infections.
Introduction
It is well known that nutrients can actively affect the maintenance and optimal functioning of immune cells [1]. Long-chain polyunsaturated fatty acids (LCPUFAs) have a very special role in this process because they participate in controlling chronic and acute inflammations. The metabolites of both omega-3 (ω3) and omega-6 (ω6) play an important role in the synthesis of different mediators, such as prostaglandins, leukotrienes, thromboxanes, protectins, and resolvins [1]. Particularly ω3 LCPUFAs could notably help improve the resolution of the inflammatory process and may also interact at different stages of viral infections. In this narrative literature review, we provide information on LCPUFAs and the possible effects of these dietary compounds in promoting better immune function and, therefore, as a co-adjuvant treatment to decrease the severity of disease among those who have been diagnosed with respiratory tract infections.
Among the respiratory viruses, particular attention has been paid to coronavirus disease 2019 (COVID-19), identi\fied for the first time in December 2019 in Wuhan, China. Because of its rapid spread worldwide and the increased infection rate, the WHO declared it a pandemic in March 2020 [2]. In 10-15% of all cases, COVID-19 is complicated by severe pneumonia requiring hospitalization, with a high risk of developing acute respiratory distress syndrome, which can lead to intensive care unit placement of the pa\tient and is often lethal. Other patients may remain asymp\tomatic even if they test positive for the virus [3]. In this context, due to its highly infectious nature and alarming mortality rate, every effort is focused on prevention and treatment to alleviate the suffering of COVID-19 patients, including the immune response.
The Immune System and LCPUFAs
The immune system is a network of biological processes with the main aim to protect the organism from pathogenic organ\isms and, therefore, from disease. Overactivity of the immune system can display many forms, including an excessive reaction to allergens or autoimmunity, while underactivity of the immune system (immunodeficiency) can be due to primary immunodeficiency diseases or can be the result of other conditions, such as cancer, immunosuppressants, and HIV/AIDS. These conditions increase the susceptibility to other infections.
LCPUFAs are an essential component of immune cells, and their relationships are an ongoing matter of research. It has been reported that in lymphocytes, monocytes, and neutrophils, arachidonic acid (ARA) constitutes about 20% of total fatty acids, while ω3 eicosapentaenoic acid (EPA) and docosahexaenoic acid (DHA) constitute 1 and 2.5%, respectively [4].
ω6/ω3 Equilibrium
LCPUFAs are synthesized by elongation and desaturation of the carbon chain from the essential PUFAs: linoleic acid (LA) for the ω6 series and alpha-linolenic acid (ALA) for the ω3 series [5]. The metabolic pathway consists of successive carbon chain elongation and desaturation steps as illustrated in Figure 1. Due to the different roles and effects of the ω6 and ω3 series, it is important to understand the effect of the ω6 to ω3 ratio on the immune system, in particular the ratio of LA/ALA.
LA, defined as an essential fatty acid in mammals because of their inability to synthesize it, is common in the human diet, being widely distributed in foods [6]. In many vegetable oils, it represents more than 50% of the lipid content; high amounts of LA are also present in nuts, while lower levels are found in cereals (more in whole grains), legumes, some meats, eggs, and dairy products [6]. LA has been reported to be the substrate for CYP450 enzymes, leading to the formation of linoleic epoxides 9,10-epoxyoctadecenoic acid (9,10-EpOME), and 12,13-epoxyoctadecenoic acid (12,13-EpOME) known as leukotoxin and isoleukotoxin [7]. The epoxides are then metabolized by the soluble epoxide hydrolases into the dihydroxy derivatives 9,10-DiHOME and 12,13-DiHOME. DiHOMEs may play a dual role in inflammation, stimulating neutrophil chemotaxis at low concentrations, while inhibiting neutrophil respiratory burst at higher concentrations [8]. Like LA, ALA is defined as an essential fatty acid in mammals [9], and its principal diet sources are nuts, leafy vegetables, and seed oils. After absorption, it can be catabolized into longer-chain and more unsaturated fatty acids, such as EPA and DHA, but, like LA conversion into ARA within the ω6 series, the endogenous production of ALA derivatives is low in humans.
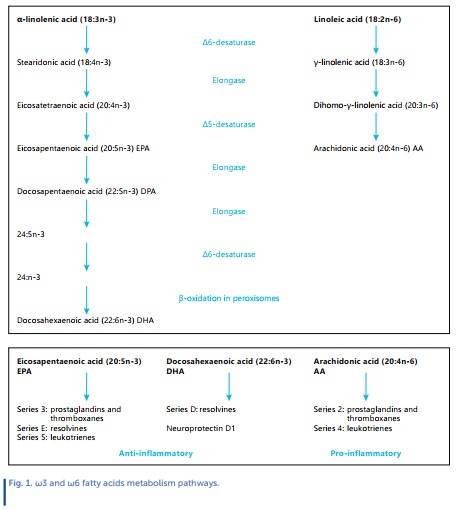
Arachidonic Acid and Its Metabolites and the Immune System
ARA is the main ω6 product and is present esterified to the 2-position in membrane phospholipids [10]. Upon release from membrane phospholipids by the activity of the cytosolic phospholipase A2 (PLA2), ARA is enzymatically metabolized by several oxygenases into eicosanoids, a large family of mostly proinflammatory molecules. The main ARA metabolites involved in the immune system are prostaglandin E2 (PGE2), leukotriene B4 (LTB4), and thromboxane A2 (TXA2). PGE2 inhibits T-cell and NK-cell proliferation, as well as interferon-y (IFN-y) and interleukin-12 (IL-12) production after binding cell surface receptors [11, 12]. PGE2 also inhibits B-cell activation secondary to IL-4 stimulation in a specific manner and enhances IgE and IgG1 production [13].
LTB4 enhances T-cell recruitment, inhibits de novo induced Tregs generation, and increases IL-17 cytokine production during T-cell differentiation. Also, it regulates the migration of various lymphoid-derived cell types [14]. TXA2 inhibits naive T-cell proliferation. Moreover, it inhibits T-cell interaction with dendritic cells and increases mature T-cell proliferation and activation [11].
Regarding the role of ARA and SARS-CoV-2, two recent reviews resume some pivotal points. Ripon et al. [15] describe the role of the ARA metabolic cascade during the cytokines storm due to virus infection, concluding with the recommendation to use bioactive lipids, nonsteroidal anti-inflammatory drugs, steroids, cell phospholipase A2 (cPLA2) inhibitors, and specialized pro-resolving mediators to treat COVID-19 disease, while Das [16] explains and supports the use of ARA to counteract SARS-CoV-2 due to its active role against viruses and to reduce inflammation.
ω3EPA, DHA, and Their Metabolites and the Immune System
ω3 LCPUFAs act to inhibit the inflammatory response both in a direct and indirect pathway: DHA reduces endoplasmic reticulum stress and reactive oxygen species production in mitochondria, inhibits Toll-like receptor activation, and upregulates cytoprotective proteins, intracellular antioxidants, and anti-inflammatory and detoxifying enzymes via the activation of nuclear factor erythroid 2-related factor 2 (NRF2). Both DHA and EPA regulate the inflammation response acting on the expression of oxidized low-density lipoprotein receptor 1, plasminogen activator inhibitor 1, TXA2 receptor, vascular cell adhesion molecule-1, monocyte chemoattractant protein-1, and intercellular adhesion molecule 1 [17]. EPA, docosapentaenoic acid, and DHA are the precursors of several oxygenated lipids. The resulting compounds have been collectively described as a novel genus of specialized pro-resolving lipid mediators and are endowed with potent anti-inflammatory, pro-resolution activities within the immune system. This molecular family includes D- and E-series resolvins, protectins, maresins [18, 19], and maresin conjugates in tissue regeneration, which have been proved effective in limiting inflammation and accelerating the physiological resolution of the inflammatory response [18, 20].
Methods
A nonsystematic review of the literature was initially performed in May 2021 and then updated in November 2021. The search was carried out via PubMed (www.ncbi.nlm.nih.gov/pubmed), Embase (www. emabase.com), Web of Science (www.isiknowledge.com), and the Cochrane library (www.cochranelibrary.com). In this narrative review, we aim to identify whether LCPUFA supplementation may be effective in protecting the population against respiratory tract infections. Only human studies reported in English were considered. Letters to the editor, abstracts, and proceedings from scientific meetings were excluded from the analysis. Two authors (V.C. and A.M.) independently selected the articles, retrieved and assessed the potentially relevant ones. Eligible were clinical trials on LCPUFA supplementation (intervention) and respiratory tract infection (outcome), including participants of all ages and from any region of the world. Secondary references of identified papers were also screened.
Results
A total of 8 RCTs were identified [21-28], which are detailed in Table 1. They included 4,107 subjects. Five trials were performed in a pediatric population (age 0-11 years) and 3 studies in adults (age 18-45 years). One study was conducted in Italy, 1 in Mexico, 1 in Denmark, 1 in France, 1 in Africa, 2 in the USA, and 1 multicenter study was conducted in Malaysia, the Netherlands, Poland, Portugal, and Thailand. A wide range of LCPUFA dosages and types was used for supplementation. The duration of the intervention was 4-9 months. Three studies showed a reduced incidence of upper respiratory tract infections or infective episodes in children when fed with DHA, ARA or ALA/LA, or EPA/DHA, respectively, in the first year of life [21-23].
First, Birch et al. [21] reanalyzed data from two cohorts to investigate the incidence of allergic and respiratory diseases in children with a DHA/ARA-supplemented formula (DHA/ARA as 0.32-0.36% and 0.64-0.72% of total fatty acids, respectively) during infancy. They found lower odds of developing upper respiratory infection in the DHA/ARA- supplemented group for 1 year. Second, Venuta et al. [22] designed an interventional study in children between 36 and 49 months of age to evaluate the impact of supplementation of LA (596 mg/day) and ALA (855 mg/day) on respiratory tract infections. The authors observed a reduced number of infectious episodes, days of fever, and absence from school at 120-180 days from intervention in children receiving supplementation. Malan et al. [23] supplemented 321 children aged 6-11 years with 50 mg Fe++, 420 mg DHA, and 80 mg EPA given on 4 days/week. The supplementation was associated with a 3-fold lower odds of school absence (OR: 0.30, 95% CI: 0.11, 0.82), but no associations with other outcomes were found.
Two more studies analyzed LCPUFA supplementation in formula milk. Specifically, Lapillonne et al. [24] observed that infants fed with formula containing 17 mg DHA and 34 mg ARA/100 kcal had a lower incidence and delayed onset of respiratory illnesses (bronchitis/bronchiolitis) and of their symptoms (croup, nasal congestion, cough), as well as less diarrhea requiring medical attention, when compared to infants who received formula without DHA and ARA. On the contrary, Chatchatee et al. [25] in an international multicenter interventional study failed to demonstrate a reduced risk of infection in 767 healthy children fed with growing-up milk with the addition of 1.2 g/100 mL of short-chain galacto- oligosaccharides/long-chain fructo-oligosaccharides (sc- G0S/ICFOS) (9:1) and 19.2 mg/100 mL of ω3 LCPUFAs (EPA + DHA, 4:6).
Two studies involved pregnant women. Imhoff-Kunsch et al. [26] studied the effect on infant morbidity of 400 mg of DHA taken daily by mothers in the prenatal period. This double-blind randomized placebo-controlled trial demonstrated a lower occurrence of cold symptoms in the DHA group with respect to placebo at 1 and 3 months of age (37.6 vs. 44.6% and 37.8 vs. 44.1%, respectively). Moreover, a shorter duration of nasal secretion, fever, and difficulty breathing at 6 months (RR: 0.87, 95% CI: 0.77-0.98; RR: 0.80, 95% CI: 0.66-0.98; and RR: 0.46, 95% CI: 0.24-0.87, respectively) was reported. Another trial with the same design by Bisgaard et al. [27] studied 736 pregnant women supplemented with 2.4 g/day of ω3 LCPUFA (55% EPA and 37% DHA) in the third trimester and concluded that the infants of supplemented mothers had a reduced risk of both wheeze or asthma and of infections of the lower respiratory tract. Lastly, only 1 RCT investigated the effect of 2 g/day of conjugated LA in a population aged 18-45 years and showed no consistent effects on subjects affected by human rhinovirus colds.
Discussion and Conclusion
There are few clinical trials on LCPUFA supplementation to prevent and manage respiratory tract infections, but available results point out a potential role of LCPUFA supplementation in preventing respiratory tract infections. This positive impact on reducing diseases of the respiratory tract is highlighted also by data from observational studies as reported by Pastor et al. [29]. They found a decreased incidence of bronchiolitis/ bronchitis, upper airway infection, and rhinitis in infants fed a formula supplemented with DHA/ARA compared with infants fed with lower levels of DHA/ARA supplementation or no supplementation at all [29]. The mechanisms underlying the effect of LCPUFAs remain largely unknown. On the one hand, it is possible that the reduction of inflammation of the respiratory airways might prevent symptomatic disease. In that case, subjects such as those with allergic asthma might have a significant benefit from supplementation with LCPUFA [21]. On the other hand, the effect of LCPUFA supplementation was also observed in other subjects at risk of infections, such as those receiving chronic iron supplementation [23].
Finally, the potentially beneficial effect was confirmed in healthy subjects, too. These data point out that the benefit of LCPUFA supplementation is not limited to subjects with chronic inflammation and might represent a promising strategy for preventing viral diseases. However, we could not identify any trial investigating the effect of LCPUFA in the prevention or treatment of respiratory tract infection due to SARS- CoV-2 infection. It is important to note that the strength of available evidence is also limited by important factors. The included trials are heterogenous with respect to the cohorts considered, the type of supplementation, and the duration. While the current results add to the understanding of the potential health benefits of LCPUFAs in infants' diet, our review has some important limitations. First, the results are poorly comparable, and a formal evaluation of the studies' quality was not performed. For instance, the lack of positive conclusions in Chatchatee et al.'s [25] study is probably due to the nonhomogeneous cohort with significantly different diet attitudes. Another limitation is the small cohort size of 2 studies: 38 subjects fed with DHA in the study of Birch et al. [21], and 20 subjects considered by Venuta et al. [22]. Different definitions of respiratory illness were used in the trials, and the incidence of upper respiratory tract infection was measured at different time points. Moreover, the methods to ascertain respiratory infections were not consistent among studies. In 4 studies, the respiratory illness was a secondary outcome, so that the sample size estimates for respiratory tract infection differences between the groups were not calculated. No adjustments for potential confounders were performed in most of the trials, and because multiple secondary outcomes were tested, the type II error rate could have been inflated. The study of Lapillonne et al. [24] was observational and not randomized. Moreover, in the same study regarding milk formula, the use of DHA/ARA formula may have been more prevalent in families who were of a higher socioeconomic status, which may have introduced a recruitment bias. A further weakness concerns the presence of small study effects, including, but not limited to, publication bias, which was not possible to assess.
The possibility to reduce the impact of diseases like COVID-19, and other future similar diseases, with a dietary change and/or nutritional supplementation may be useful for several reasons: less stress on the national health system and the possibility to avoid drugs' side effects. It is also difficult to translate these considerations, also if positive, to the COVID-19 scenario, since it is a complex disease that involves several biochemical and physiological mechanisms [29]. This review highlights the potential association of LCPUFAs with respiratory infection, possibly in infants, children, and adults. The immunological scientific evidence is compelling, but it is difficult to draw firm conclusions from the few clinical studies. Due to the narrative nature of this review, it is not possible to draw conclusions on whether different supplementations of fatty acids, derived from either infant formulas or supplementation of the infant's mother during pregnancy, may provide a protective effect on the incidence of respiratory infections or on symptoms. On the other hand, this review highlights that new randomized controlled trials designed to address the impact of LCPUFAs on respiratory infections are needed. In particular, the influence of perinatal ω3 PUFA nurture on infant immune function should be studied. Finally, additional studies including infants exposed to the same supplementation of fatty acids from birth might allow to drive firm conclusions on the role of LCPUFAs in the immune function of infants.
Conflict of Interest Statement
The writing of this article was supported by Nestlé Nutrition Institute and the authors declare no other conflicts of interest.
Author Contributions
V.C., S.T., and A.M. drafted the manuscript; C.A. and G.P.M. supervised the work and gave technical support on data interpretation; V.C. arranged the references; A.M. and M.L.S. proofread the manuscript. All authors contributed significantly to the paper and agreed on the manuscript in its current form. All authors have read and agreed on the published version of the manuscript.
References
1 Mazzocchi A, De Cosmi V, Risé P, Milani GP, Turolo S, Syrén ML, et al. Bioactive Compounds in Edible Oils and Their Role in Oxidative Stress and Inflammation. Front Physiol. 2021 Apr;12:659551.
2 Hathaway D 3rd, Pandav K, Patel M, Riva-Moscoso A, Singh BM, Patel A, et al. Omega 3 fatty acids and COVID-19: a comprehensive review. Infect Chemother. 2020 Dec;52(4):478-95.
3 Guo YR, Cao QD, Hong ZS, Tan YY, Chen SD, Jin HJ, et al. The origin, transmission and clinical therapies on coronavirus disease
2019 (COVID-19) outbreak - an update on the status. Mil Med Res. 2020 Mar;7(1):11.
4 Calder PC. The relationship between the fatty acid composition of immune cells and their function. Prostaglandins Leukot Essent Fatty Acids. 2008 Sep-Nov;79(3-5):101-8^
5 Agostoni C, Bruzzese MG. [Fatty acids: their biochemical and functional classification]. Pediatr Med Chir. 1992 Sep-Oct;14(5):473-9.
6 Marangoni F, Agostoni C, Borghi C, Catapano AL, Cena H, Ghiselli A, et al. Dietary linoleic acid and human health: focus on cardiovascular and cardiometabolic effects. Atherosclerosis. 2020 Jan;292:90-8.
7 Hildreth K, Kodani SD, Hammock BD, Zhao L. Cytochrome P450- derived linoleic acid metabolites EpOMEs and DiHOMEs: a review of recent studies. J Nutr Biochem. 2020 Dec;86:108484.
8 Thompson DA, Hammock BD. Dihydroxyoctadecamonoenoate esters inhibit the neutrophil respiratory burst. J Biosci. 2007 Mar;32(2):279-91.
9 Das UN. Essential fatty acids: biochemistry, physiology and pathology. Biotechnol J. 2006 Apr;1(4):420-39.
10 Chilton FH, Murphy RC. Remodeling of arachidonate-containing phosphoglycerides within the human neutrophil. J Biol Chem. 1986 Jun;261(17):7771-7.
11 Harizi H, Gualde N. The impact of eicosanoids on the crosstalk between innate and adaptive immunity: the key roles of dendritic cells. Tissue Antigens. 2005 Jun;65(6):507-14.
12 Nataraj C, Thomas DW, Tilley SL, Nguyen MT, Mannon R, Koller BH, et al. Receptors for prostaglandin E(2) that regulate cellular immune responses in the mouse. J Clin Invest. 2001 Oct;108(8):1229-35.
13 Roper RL, Phipps RP. Prostaglandin E2 and cAMP inhibit B lymphocyte activation and simultaneously promote IgE and IgG1 syn-thesis. J Immunol. 1992 Nov;149(9):2984-91.
14 Goodarzi K, Goodarzi M, Tager AM, Luster AD, von Andrian UH. Leukotriene B4 and BLT1 control cytotoxic effector T cell recruitment to inflamed tissues. Nat Immunol. 2003 Oct;4(10):965-73.
15 Ripon MA, Bhowmik DR, Amin MT, Hossain MS. Role of arachidonic cascade in COVID-19 infection: A review. Prostaglandins Other Lipid Mediat. 2021 Jun;154:106539.
16 Das UN. Bioactive Lipids in COVID-19 - Further Evidence. Arch Med Res. 2021 Jan;52(1):107-20.
17 Yamagata K. Docosahexaenoic acid regulates vascular endothelial cell function and prevents cardiovascular disease. Lipids Health Dis. 2017 Jun;16(1):118.
18 Serhan CN, Levy BD. Resolvins in inflammation: emergence of the pro-resolving superfamily of mediators. J Clin Invest. 2018 Jul;128(7):2657-69.
19 Serhan CN, Gupta SK, Perretti M, Godson C, Brennan E, Li Y, et al. The Atlas of Inflammation Resolution (AIR). Mol Aspects Med. 2020 Aug;74:100894.
20 Dalli J, Sanger JM, Rodriguez AR, Chiang N, Spur BW, Serhan CN. Identification and Actions of a Novel Third Maresin Conjugate in Tissue Regeneration: MCTR3. PLoS One. 2016 Feb;11(2):e0149319.
21 Birch EE, Khoury JC, Berseth CL, Castaneda YS, Couch JM, Bean J, et al. The impact of early nutrition on incidence of allergic manifestations and common respiratory illnesses in children. J Pediatr. 2010 Jun;156(6):902-906.e1.
22 Venuta A, Spanò C, Laudizi L, Bettelli F, Beverelli A, Turchetto E. Essential fatty acids: the effects of dietary supplementation among children with recurrent respiratory infections. J Int Med Res. 1996 Jul-Aug;24(4):325-30.
23 Malan L, Baumgartner J, Calder PC, Zimmermann MB, Smuts CM. n-3 Long-chain PUFAs reduce respiratory morbidity caused by iron supplementation in iron-deficient South African schoolchildren: a randomized, double-blind, placebo-controlled intervention. Am J Clin Nutr. 2015 Mar;101(3):668-79.
24 Lapillonne A, Pastor N, Zhuang W, Scalabrin DM. Infants fed for-mula with added long chain polyunsaturated fatty acids have reduced incidence of respiratory illnesses and diarrhea during the first year of life. BMC Pediatr. 2014 Jul;14(1):168.
25 Chatchatee P, Lee WS, Carrilho E, Kosuwon P, Simakachorn N, Yavuz Y, et al. Effects of growing-up milk supplemented with pre- biotics and LCPUFAs on infections in young children. J Pediatr Gastroenterol Nutr. 2014 Apr;58(4):428-37.
26 Imhoff-Kunsch B, Stein AD, Martorell R, Parra-Cabrera S, Romieu I, Ramakrishnan U. Prenatal docosahexaenoic acid supplementa¬tion and infant morbidity: randomized controlled trial. Pediatrics. 2011 Sep;128(3):e505-12.
27 Bisgaard H, Stokholm J, Chawes BL, Vissing NH, Bjarnadottir E, Schoos AM, et al. Fish Oil-Derived Fatty Acids in Pregnancy and Wheeze and Asthma in Offspring. N Engl J Med. 2016 Dec;375(26):2530-9.
28 Peterson KM, O'Shea M, Stam W, Mohede IC, Patrie JT, Hayden FG. Effects of dietary supplementation with conjugated linoleic acid on experimental human rhinovirus infection and illness. An- tivir Ther. 2009;14(1):33-43.
29 Pastor N, Soler B, Mitmesser SH, Ferguson P, Lifschitz C. Infants fed docosahexaenoic acid- and arachidonic acid-supplemented formula have decreased incidence of bronchiolitis/bronchitis the first year of life. Clin Pediatr (Phila). 2006 Nov;45(9):850-5.
30 Nayeri ND, Nadali J, Divani A, Basirinezhad MH, Meidani M. Clinical manifestation, laboratory and radiology finding, treatment and outcomes of COVID-19: A systematic review and meta-analysis. J Res Med Sci. 2021;26:41.